
RESEARCH
PLANT DEVELOPMENT
To understand the self-organization of plant architecture we construct the multilevel computer models that integrate quantitative experimentally-derived data. Our computer models include intercellular transport of hormones, polarity determinants, and dynamics of cell growth among other components. We apply those models to understand early embryogenesis, organogenesis, leaf venation patterning, organ bending, and root patterning. We collaborate closely with experimentalists in a feedback dependent manner to improve models and propose further experiments that ultimately led to a better understanding of plant development.
Currently, we combine new methods from the computer graphics field to efficiently resolve the mechanics of plant tissue growth in the combination with biochemical processes. We develop supercomputing pipelines to run hundreds of simulations simultaneously to scan the parameter space for possible phenotypes using customized MorphoGraphX (https://www.mpipz.mpg.de/MorphoGraphX/) software packages
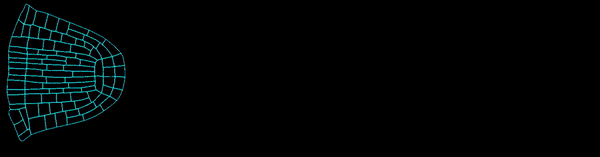
SYNTHETIC CELL COMMUNICATION SYSTEMS
SYNCHRONIZATION THROUGH HORMONE RHYTHMS
Using synthetic biology paradigm we reconstruct minimal genetic circuits consisting of natural and synthetic phytohormone signaling components wired in feedback loops in the baker's yeast Saccharomyces cerevisiae. We study circuits under a precisely controlled environment on the microfluidics lab-on-the-chip devices using time-lapse epifluorescent microscopy. A fluorescent output of circuits is monitored in real-time through automated acquisition and image processing algorithms. We aim to understand design principles behind phytohormone circuit dynamics as well as to build new regulatory modules based on orthogonalities of signaling components. We currently focus on putative circuits that steer cyclic organ initiations in Arabidopsis and new synthetic circuits integrating components of microbial origin. Our key goal is to construct robust, orthogonal synthetic phytohormone crosstalk systems that can be used in plants and other multicellular organisms.
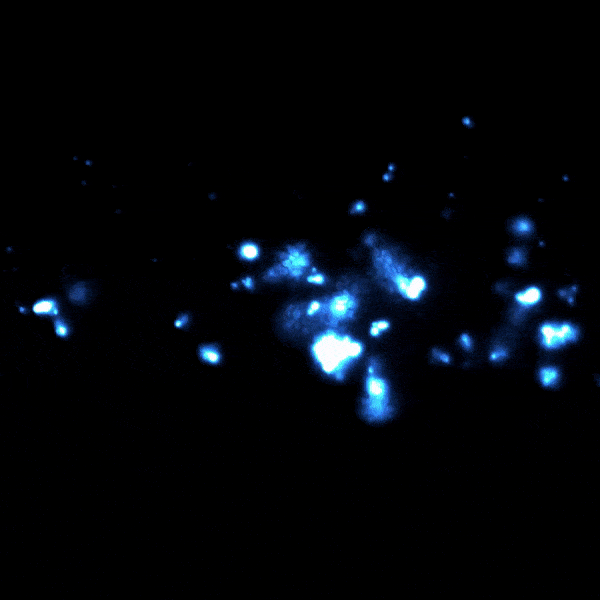
Sensing environmental changes is key for the survival of any living organisms, from the most primitive bacteria to most complex multi-cellular organisms. During evolution, both uni- and multi-cellular organisms have developed a wide range of receptors that are capable to detect various signals such as; the presence of pathogens agents, changes of temperature, luminosity among others. We can differentiate two prominent groups of receptors; those that are places in the membrane and are responsible for sensing extracellular signals, and those that are localized in the cytoplasm, nucleus or other organelles, responsible for the perception of intracellular signals.
Many of these receptors operate in pairs as homo- or hetero-dimers and transfer a signal from the environment to the cell interior that depends on the presence of a particular chemical or physical stimulus. Inherent modularity of receptors allows bioengineering approaches to construct synthetic hybrid receptors with exchangeable sensory parts.
Our general approach is to build novel synthetic perception systems at the plasma membrane of eukaryotic cells and use these systems to communicate different cell populations via engineered ligands to create synthetic eukaryotic microbiomes.
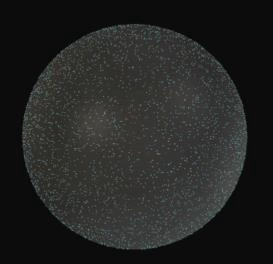
LOW-COST MICROFLUIDICS PROTOTYPING
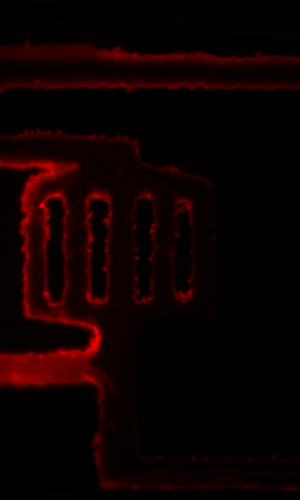
We develop low-cost techniques and methods to prototype microfluidic devices and use them to perform high-throughput simultaneous experiments. Our customized microfluidics platform combines the fully automated microscopy platform with software that controls media switching. This platform allows currently for use of up to five independent microfluidic devices in each experiment under a precisely controlled environment.